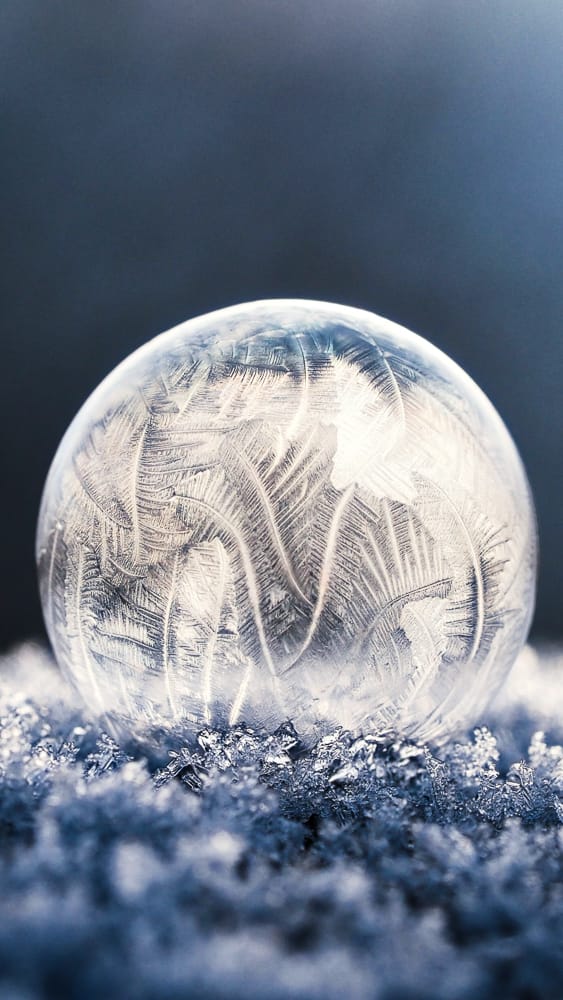
Bubble Crystallization
The patterns captured on the surface of a frozen water bubble reveal the intricate process of crystallization, a natural transformation of water into ice.
When a thin bubble of pure water is exposed to freezing temperatures, it undergoes a rapid yet stunning physical change driven by the unique properties of water molecules. Crystallization begins when the temperature drops below freezing, and the supercooled water within the bubble transitions from a liquid to a solid state.
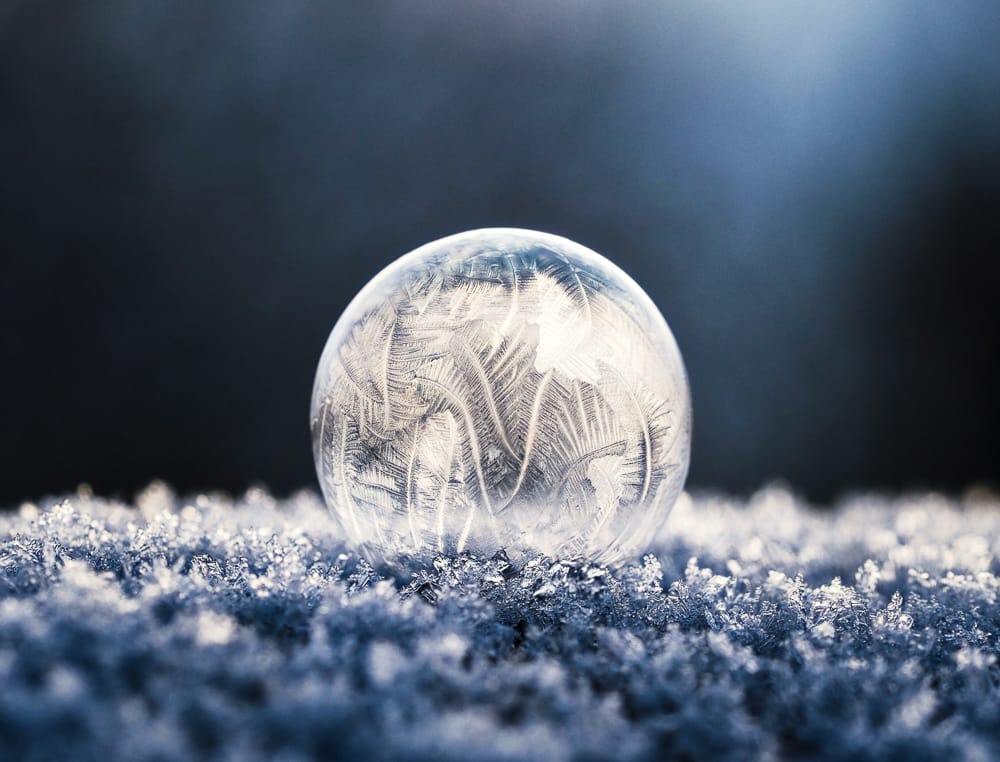
This transformation starts at nucleation points—tiny imperfections on the bubble’s surface or within the water itself. These points act as seeds where the first ice crystals form. Once nucleation begins, the surrounding water molecules align themselves into an ordered crystalline structure due to hydrogen bonding, a defining feature of water’s molecular behavior.
As the ice crystals grow, they develop intricate, dendritic (tree-like) patterns. This occurs because water freezes anisotropically, meaning that the rate of crystal growth differs depending on the molecular orientation. The feather-like structures arise as the ice expands more rapidly along certain axes, creating the ornate and symmetrical designs visible in the frozen bubble.
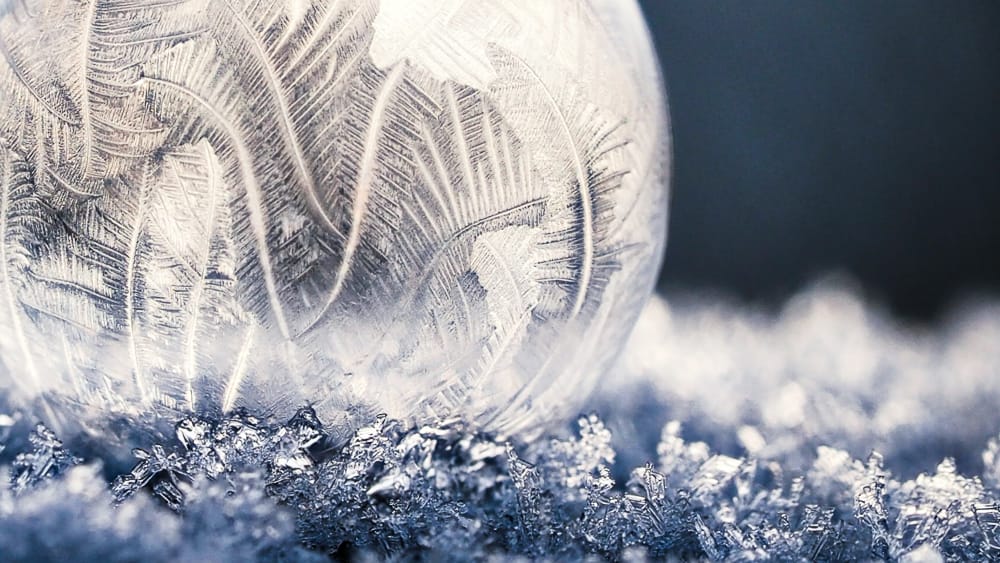
The ambient temperature, humidity levels, and even the stillness of the air all influence the formation process. In calm conditions, the crystals grow symmetrically, forming delicate, fern-like patterns. In contrast, slight air movement or fluctuations in temperature can disrupt the process, introducing asymmetries or irregularities.
As the water layer cools rapidly, it freezes before the bubble can collapse. The tension in the bubble’s thin membrane allows it to maintain its spherical shape long enough for the crystallization process to complete, resulting in a frozen globe of intricate ice artistry.
Learn more from our sources: Libbrecht, K. G. (2005). The Physics of Ice Crystals and Petrenko, V. F., & Whitworth, R. W. (1999). Physics of Ice.